Research Area
- Properties of Sulfonated Poly(phenylene) Ionomers
- Ab Initio Simulations of Proton Transport in Confined Environments
- Degradation Mechanisms of PFSA Membranes
- Transport of Protons and Water Through the Ionomer-Membrane Interface
- Effects of Side Chain Connectivity on Proton Transfer in 3M Perfluorosulfonic Acid Membranes
- Simulations of Proton Transfer in Ionomers with Multiple Acidic Groups
- Dissipative Particle Dynamics Simulations of PFSA Morphology
- Dielectric Response of Hydrated PFSA Membranes
Properties of Sulfonated Poly(phenylene) Ionomers
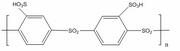
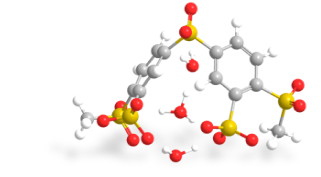
A novel class of sulfonated poly(phenylene) ionomers consisting of aromatic rings and sulfone units (-SO2-) have recently been synthesized and characterized. These ionomers show considerable thermooxidative and hydrolytical stability due to the highly electron-deficient phenyl rings and electron-accepting sulfone units in the backbone. At low relative humidity and high temperatures, sulfonated poly(phenylene) sulfone (sPSO2) ionomers possess from 5 to 7 times higher proton conductivity than observed in Nafion. We are trying to understand: 1) Proton transfer in micro-hydrated polysulfone membrane by use of electronic structure calculation (DFT) methods; 2) Morphology of polysulfone membranes as a function of hydration by performing Dissipative particle dynamics (DPD) simulations; 3) Experimental characterization of polysulfone polymer using Transmission Electron Microscope (TEM).
Ab Initio Simulations of Proton Transport in Confined Environments
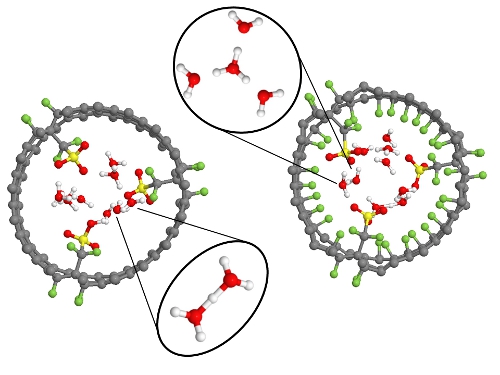
Single walled carbon nanotubes (CNT) were functionalized with perfluorosulfonic acid (-CF2SO3H) groups and hydrated with water molecules (1 and 3 H2O/SO3H) to investigate proton dissociation and transport in simplified proton exchange membranes under conditions of minimal hydration. The systems were studied using ab initio molecular dynamics (AIMD), which does not require a priori assumptions about proton dissociation and hydration. The influence of the hydrophobic environment was studied by comparing proton mobilities with and without fluorine atoms attached to the CNT walls. The AIMD trajectories showed that dissociation of the acidic proton was increased as sulfonic acid density increased, however, greater densities also increased trapping of the dissociated proton. The fluorine atoms accepted hydrogen bonds from the water molecules, stabilized hydrogen bonding, and enhanced proton dissociation. The CNT systems with bare walls exhibited a propensity for Zundel cation (H5O2+) formation, while the fluorinated systems favoured the hydronium cation (H3O+).
Degradation Mechanisms of PFSA Membranes
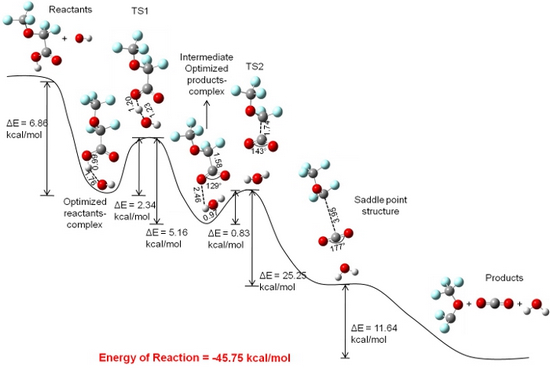
PFSA membranes such as Nafion® and short sidechain membranes are widely used in the polymer electrolyte membrane (PEM) fuel cells due to their good proton conductivity in hydrated form. These membranes have poly-tetrafluoroethylene backbone, and sidechains have ether-linked perfluorinated chain with sulfonic acid end group. Backbone provides physical and chemical stability in the membrane and sidechain provides an extra proton when the membrane is sufficiently hydrated. In the operating PEM fuel cell, there is an evidence of the formation of hydrogen peroxide (H2O2) due to crossover of oxygen gas and its reaction with atomic hydrogen at anode. H2O2 can further break into hydroxyl and hydrogen peroxyl radials and can form a strong oxidizing medium. Hydroxyl radial has the highest reduction potential i.e. the most reactive oxidizing agent. We have studied the degradation of backbone and sidechain of the PFSA membranes in the presence of the hydroxyl radical using an ab initio calculation on Guassian 03 suites of program. B3LYP method alongwith 6-311G**(d, p) basis set was employed for the optimization of reactants and products, the determination of transition states of the reactions, and for an intrinsic reaction coordinate (IRC) calculation at the transition state. Our results establish the backbone degradation mechanism by unzipping mechanism proposed by Curtin’s group. Results also show that the sidechains degradation starts with the breakage of the C-S bond.
Transport of Protons and Water Through the Ionomer-Membrane Interface
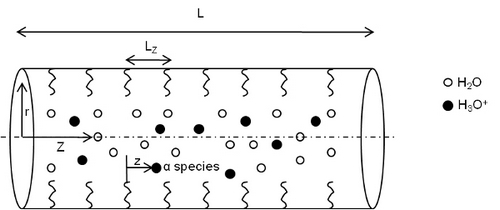
An interface between membrane and ionomer is formed in membrane electrode assembly. Nafion® solution is used as ionomer which consists of sulfonic acid group. In this study, the pores in the interface are considered as cylinders, and sulfonic anions are distributed on the wall. The transport of proton and water through the pore is due to convection and change in the chemical potential along the pore length. Total chemical potential of the species in the pore is the result of species-species interaction and their interaction with sulfonic anions on the pore wall. The chemical potential energies were macroscaled and were applied to Liouville equation to obtain the evolution equations for density and velocity of the water and hydronium ions in the pore. These equations were numerically solved to get density and velocity profiles, which were used to get the conductivity of proton in the pore of the interface. The results show that the conductivity is in good agreement with the experimental results.
Effects of Side Chain Connectivity on Proton Transfer in 3M Perfluorosulfonic Acid Membranes
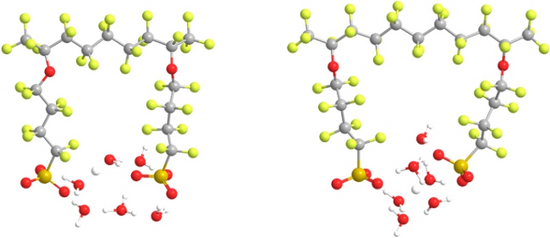
The role connectivity of adjacent side chain acid groups of 3M perfluorosulfonic acid (PFSA) membranes has on affecting proton dissociation and transfer was explored through a consideration of oligomeric fragments with different poly(tetrafluoroethylene) (PTFE) backbone segments separating the side chains. Electronic structure calculations were performed at the B3LYP/6-311G** level of theory on fragments with chemical formula: CF3CF(–O(CF2)4SO3H)(CF2)nCF(–O(CF2)4SO3H)CF3, where n = 5 or 7, corresponding to membrane equivalent weights of 590 and 690 g/mol, respectively. Fully optimized structures of these fragments with and without the addition of water molecules revealed that connectivity of the SO3H groups through hydrogen bonding is critical for proton dissociation as well as the state of the dissociated proton. Proton dissociation was first observed in the fragment with an EW 590 at a water content of only 1 H2O/SO3H with dissociation of the second acidic proton occurring at a hydration as low as 1.5 H2O/SO3H. The system with greater separation of the side chains (EW 690), however, did not exhibit proton dissociation of the first and second acidic protons until four and five water molecules were added in geometries with fully extended backbones, respectively, as the side chain separation was too great to facilitate the cooperative interaction that promotes proton dissociation at low hydration in membranes of this type.
Simulations of Proton Transfer in Ionomers with Multiple Acidic Groups
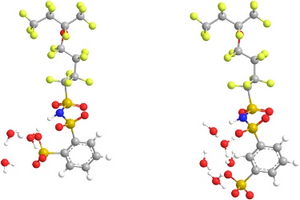
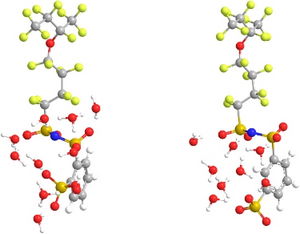
Proton Exchange Membrane (PEM) fuel cells offer tremendous potential as clean alternative energy conversion systems. Critical to these devices is the proton-conducting polymer electrolyte membrane. It is widely known that proton conductivity in many of these membranes strongly depends on the level of hydration. However, a more complete understanding of the molecular features of proton transfer within these membranes is needed for further utilization of PEMFCs’ potential. The roles local hydration and protogenic group separation have in affecting proton dissociation in oligomeric fragments of 3M imide based ionomers at minimal hydration levels were examined through ab initio electronic structure calculations. The ionomers in consideration are structural isomeric imide based fragments consisting of a polytetrafluoroethylene (PTFE) backbone with pendant side chains containing multiple and distinct acid groups (with chemical formula CF3CF2CF(-O(CF2)4SO2(NH)SO2C6H4SO3H)CF3 with the sulfonic acid group located in either the meta or the ortho position). Fully optimized structures of these fragments with and without the addition of water molecules at the B3LYP/6-311G** level revealed that protogenic group separation and local hydration are key contributors to proton dissociation in these membranes. Specifically, charge delocalization through hydrogen bonding between protogenic groups promotes proton dissociation at low hydration. It was found that proton dissociation in the system with the sulfonic acid in the ortho position occurs at a water content of only 1.5 H2O/protogenic group as the location of the sulfonic acid group on the phenyl ring allowed for hydrogen bonding between the sulfonic acid and an oxygen from the nearest sulfonyl group. Steric hindrances in the meta bis acid system prevent this hydrogen bonding, and, hence, dissociation of a single acidic proton in the meta bis acid system was not observed until five explicit water molecules (i.e. 2.5 H2O/protogenic group). However, this interaction appears to hinder dissociation of the second acidic proton in the ortho bis acid system as a hydration of 5 H2O/protogenic group was required for dissociation while a hydration of 4 H2O/protogenic group was sufficient for dissociation of the second acidic proton in the meta bis acid system. These calculations further substantiate prior work on the importance of the interaction of protogenic groups through hydrogen bonding in the transfer and state of the dissociated protons.
Dissipative Particle Dynamics Simulations of PFSA Morphology
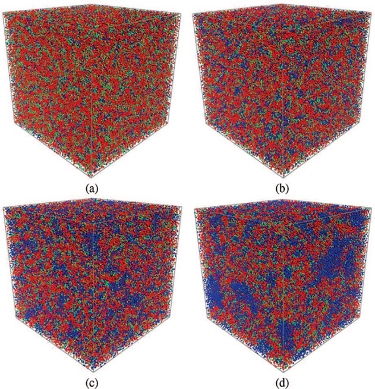
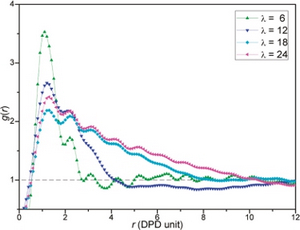
Hydrated morphologies of the SSC PFSA ionomer with an EW = 753 and MW = 18072 g/mol at water contents of: (a) 5; (b) 7; (c) 11; and (d) 16 H2O/SO3-. (Macromolecules 2009, 42, 3358-3367) Radial distribution function, G(r), of water beads for the 3M PFSA membranes with EW = 578 and MW = 45084 g/mol at various hydration levels. (Langmuir 2010, 26(17), 14308-14315)
Dielectric Response of Hydrated PFSA Membranes
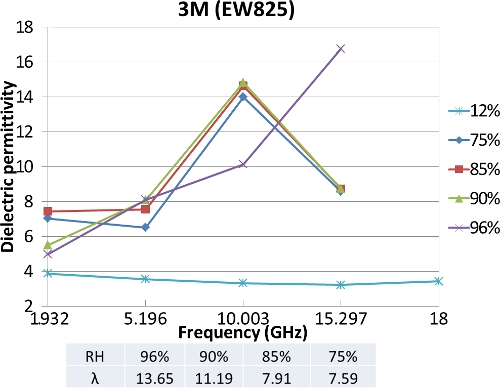
Dielectric response is used to
understand the connections between the hydrated morphology and the
local dynamics of water in polymer electrolyte membranes (PEMs) of
different equivalent weights (EW) at various hydration levels in a
controlled humidity chamber. It is widely accepted that water confined
in systems such as reverse micelles possesses different dynamics as
compared to bulk/free water. The choice to conduct measurements at
microwave frequencies (1 to 20 GHz) is due to the observation that the
principal absorption band, attributed to a Debye type relaxation of
molecular origin, in pure bulk water occurs at about 18 GHz. These
measurements can provide insights into both the state of water and
indirectly, the hydrated structure of polymer. The split-post
dielectric resonator (SPDR) technique is an accurate method for
characterization of laminar dielectrics without the use of any
electrodes. Our measurements indicate an increase in the dielectric
permittivity with water uptake in the membrane. Also we see a shift in
the peak from 10 GHz to 15 GHz as the water uptake changes from 11 to
14.
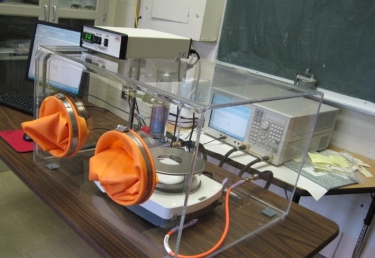
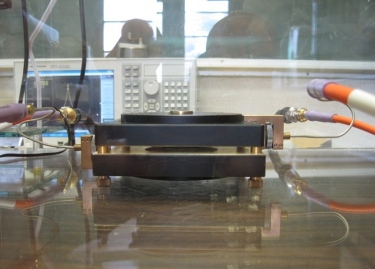